An emerging activity focusing on exploration of quantum computing and hardware development that enables quantum technologies to be experimentally developed. Developed devices are based on polariton condensates, low dimensionality electronic quantum systems, spin waves and III-Nitride based qubits aiming at high temperature quantum information systems.
Research Topics

Quantum nanoelectronics technology comes into stage mainly to give an answer to the speed and energy consumption dilemma of semiconductor nanoelectronics. MRG is working towards an in-depth investigation of the most profound manifestation of the effects of quantum coherence on macroscale and the related quantum phenomena that hold the maximal potential to become emergent new generation electronics. The main objectives tackled are the following:
- Quantum transport in quasi 1D superconductors
- Relaxation of non-equilibrium quasi particle excitations in superconductors
- Size dependence of critical temperature of superconductors
This is a new activity for the group that was officially launched in early 2018 based on the excellence of the group in both the growth of state of the art compound semiconductor hetero (nano)structures as well as in the realization of novel nanodevices.

A future quantum technological era based on the manipulation of spin, quantum superposition, and coherence is foreseen to radically transform technology, science and society. MRG investigates integrated qubits towards room temperature computing. The focus is on the development of novel concepts based on III-nitrides quantum dots exploiting specific material properties such as large bandgap and relatively long high temperature decoherence time. In addition single electron transistor (SET) structures based on 2DEG and 2DHG heterostructures are investigated by fabricating demanding top-down plasma etching and by electrostatic isolation through top metal gates, employing aggressive e-beam lithography with device scaling projected down to 10nm. Finally MRG will study the roadmap for the integration of the GaN QD technology into the FDSOI process.

The future miniaturisation of electronic circuits following Moore’s law will require the introduction of increasingly disruptive technologies to limit power consumption and optimise performance per circuit area. Spin wave computing aspires to complement and eventually replace CMOS in future microelectronics. Spin wave computing has the potential for significant power and area reduction per computing throughput while reducing cost by alleviating lithography requirements.
MRG is partner in an EU FET type funded project (“CHIRON”) that started in April 2018. As a first step towards the vision of a full spin wave computer, CHIRON envisions hybrid spin wave–CMOS circuits that can be readily integrated alongside CMOS. CHIRON will fabricate basic logic gates, such as inverters and majority gates, demonstrate their operation, and assess their performance. As transducers between the CMOS and spin wave domains in hybrid circuits, CHIRON will develop magnetoelectric and multiferroic nanoresonators, based on nanoscale bulk acoustic resonators, which bear promise for high energy efficiency and large output signal. MRG develops FBAR type nanoresonators targeting membrane thickness below 50nm and operating frequency higher than 10GHz while investigating their performance with numerous magnetostrictive materials. In addition different RF device designs and materials approaches like highly strained or doped III-nitride layers will be investigated.

Quantum simulators promise to provide unprecedented insights into physical phenomena not accessible with classical computers and have the potential to enable radically new technologies. Analog dynamical quantum simulators constitute a most promising class of architectures to fulfil the ultimate promise to devise quantum machines outperforming classical computers. Here, we devise versatile and practical platforms for dynamical simulators – making use of polariton condensate lattices and assess their computational capabilities, to probe important questions in fundamental and applied physics, ranging from technology‐relevant problems, concerning transport processes or glassy dynamics, via long‐standing challenges in the physics of non‐equilibrium phenomena. Optical lattices have attracted much attention, since they can replicate phase transition between a BEC and a Mott‐insulating state in a Bose‐Hubbard model. By experimentally modifying physical parameters, such as the periodic potential amplitude, temperature, and density, it is possible to control relative strength between on‐site interaction and hopping amplitude at will exploring the phase diagram of such systems. Such experiments have been of particular interest in the quantum information community since the experiment realizes a nearly ideal quantum simulator.
The macroscopic nature of exciton‐polariton condensates provides ease of optical access and probing of individual lattice sites normally not achievable in cold‐atom systems. Furthermore, the non‐equilibrium nature of exciton‐polariton systems is particularly attractive, because it provides the ability to prepare and study out of equilibrium many‐body phases in a natural driven dissipative setting and the ability to probe these phases in situ using optical approaches. Our recent experiments show that individual trapped polariton condensates can exhibit spontaneous magnetization, and that the spins of pairs of trapped polariton condensate can be controllably aligned. This constitutes a key step towards using trapped polariton condensates for the realization of interacting bosons in a driven‐dissipative spinor Bose‐Hubbard model. Hence, a lattice of polariton condensates is expected to model a non‐equilibrium interacting spin system with unusual properties. It is against this background that the field of polaritonics has emerged and these exotic objects are not simply an academic curiosity – rather as we discuss below we will use them as the basis for quantum simulators.
Heads
Technical Staff
Research Associates
Alumni
Infrastructure Equipment
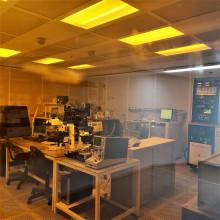
M4C setup (home made with COTS):
- AC Hall setup (magnetic field and current modulation modes, μ
- min < 0.05 cm2/Vsec)
- AC Seebeck setup (thermal modulation mode)
- AC Nernst setup (magnetic and thermal modulation modes)